With use of the NIMS original droplet epitaxy technique, we successfully fabricated ideally symmetric GaAs quantum dots and demonstrated the generation of quantum entangled photon pairs with the world-highest fidelity values (2013, Fig. 1).
Later, we developed an LED quantum dot device, which can be operated without using external lasers. Moreover, we observed single-photon emission in the telecommunication wavelengths of around 1300 and 1550 nm (2019, Fig. 2).
Recently, we focused on the development of a portable photon device that does not require a large refrigerator (Fig. 3).
Quantum Photonics Group
Group leader
Group members
AIM and GOAL
- We are aiming to create innovative photonic devices exploiting new optical functionalities that appear for advanced nanostructures.
- Study of semiconductor quantum nanostructures, their physics and applications to novel quantum light sources.
- Study of originally fabricated organic nanofibers. Their application to microoptics free from diffraction limits.
- Design of innovative quantum structures that enable artificial control of electromagnetic fields. Prediction of new quantum optical functionalities.
APPROACH
- Advanced laser spectroscopy:
We develop new spectroscopy techniques, which enable to detect optical signals from small regions, with broad spectral ranges from UV to mid-IR wavelengths. - Quantum optics:
We observe the generation of nonclassical light, which includes single photons and quantum-entangled pairs, and develop practical quantum light sources. - Theoretical analysis:
We perform electromagnetic and model analysis to investigate novel light-matter interactions in photonic nanostructures.
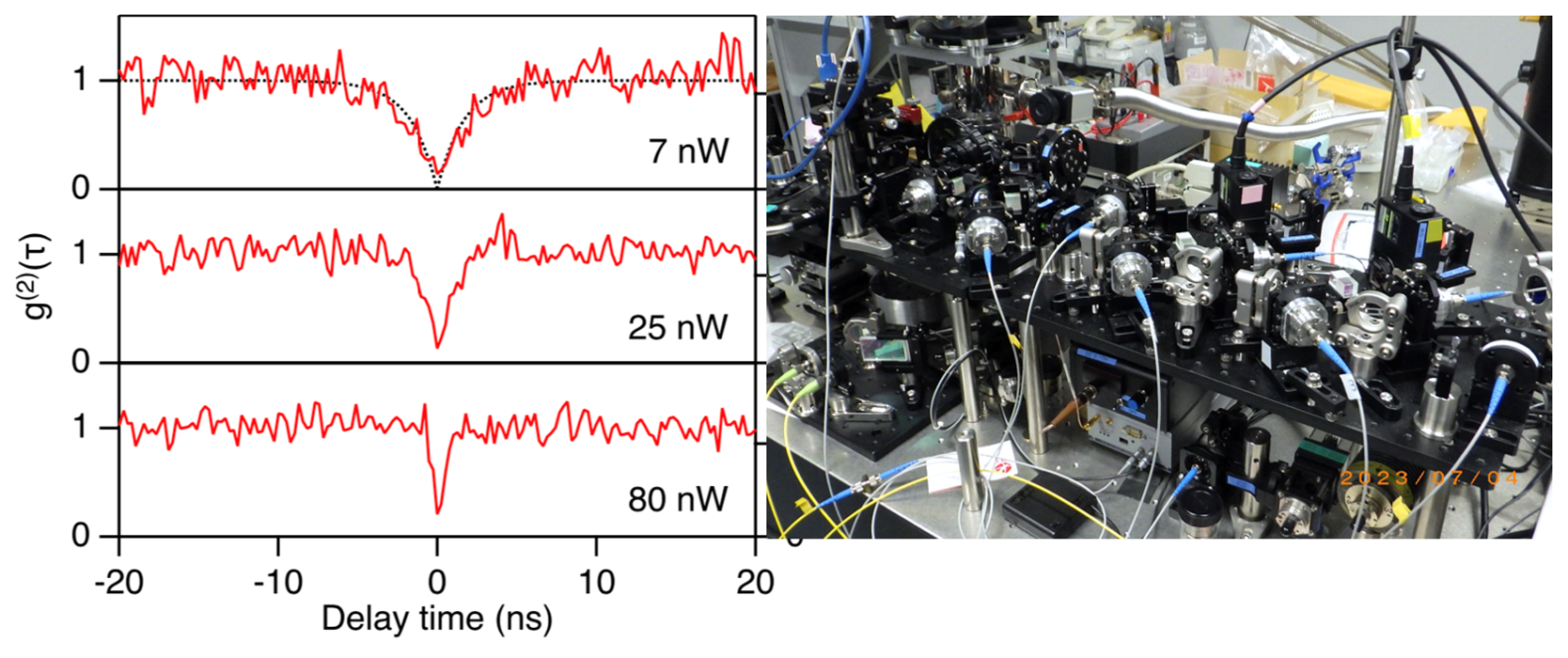
FIG 1 (Left) Anti-bunching characteristics of a quantum-dot single-photon source at a wavelength of 1.55 um.
(Right) Setup for measuring the degree of quantum entanglement.
KURODA, Takashi / Group Leader
Development of solid-state quantum light sources
Overview
Ensuring communication security has become a priority issue in modern society. Quantum key distributions or quantum communications are the ultimate secure communication method, which does not allow any information leaks. Conventional optical communications have used laser light pulses as information carriers. On the other hand, quantum communications use single photons as information carriers. Thus, the transmission distance was limited to ~100 km, which is the transmission length of light in quartz optical fibers. For longer distance communication, the development of quantum repeaters, an unexplored technology, is necessary. To achieve this, it is necessary to develop a highly efficient quantum light source that generates photons with nonlocal quantum correlation, i.e., entangled photons.
Characteristics
- Quantum dots can generate single photons and entangled photons on demand, enabling to build a large-scale quantum internet
- Use of the NIMS original fabrication techniques
- Application to LED devices and on-chip integrated circuits
Major reserch
Summary
- We have succeeded in developing the world's first high-quality quantum-dot photon source capable of generating quantum entanglement. We are promoting the following R&D towards applying our photon source to next-generation quantum communication technology.
- Wavelength tunability by using band-gap engineering
- Creation of an ideal entangled photon source that enables quantum repeaters and demonstration of quantum operations
KURIMURA, Sunao
Quantum Light Control Using Quasi-Phase-Matched Nonlinear Optical Devices
Overview
The generation and control of quantum light have attracted significant attention as novel methods for communication and measurement. The Quasi-Phase-Matched Nonlinear Optical Devices developed at NIMS operate efficiently even under low-photon density, demonstrating exceptional performance in quantum light generation and control.
NIMS has accumulated extensive knowledge in materials and device structures, advancing research and development in this field. By leveraging innovative design and fabrication techniques in nonlinear optical materials and devices, we have expanded applications in quantum light control. Our research has significantly contributed to high-bandwidth entangled-photon-pair generation and ultrafast sampling of quantum light pulses, advancing studies in quantum metrology and quantum memory.
Characteristics
By utilizing ultrafine periodic poling technology with microfabrication, we have developed high-efficiency nonlinear optical devices that can be pumped by short-wavelength lasers, such as GaN semiconductor lasers at 400 nm. The Mg:SLT crystal, developed at NIMS, plays a crucial role due to its low absorption and high damage threshold in short-wavelength range.
Compared to conventional devices without periodic poling, our devices achieve 100 times higher efficiency. This improvement allows for bandwidth expansion by smoothly varying the periodic structure using microfabrication technology. Combining material technology, periodic poling techniques, and waveguide structures, we have realized world-class efficiency.
Periodically poled nonlinear optical devices have been used to develop innovative quantum light sources, including:
- THz-bandwidth entangled photon pair sources
- Ultrafast optical sampling devices with 200 fs temporal resolution
Major reserch
At NIMS, the technological maturity of quasi-phase-matched devices based on periodic poling has enabled the design of various devices. By utilizing high-precision periodic poling technology, we have developed highly efficient devices with outstanding performance, suitable for applications such as laser displays, ultrafast communication, and optical sampling.
- Quantum Light Generation and Polarization Control
Figure 1 illustrates the process of generating low-energy entangled photon pairs from high-energy pump light. The polarization states of the output light can be selected based on the phase-matching type. The design flexibility of periodically poled devices allows for precise control over the polarization combinations of generated photons.- Type-0 phase matching offers high efficiency, making it suitable for quantum metrology and quantum teleportation.
- Type-I and Type-II phase matching provide polarization separation properties, ideal for applications in quantum communication.
At the research level, practical demonstrations in quantum metrology and quantum imaging have been conducted. Quantum communication systems leveraging this technology have already been commercialized due to their high security.
By selecting specific poling patterns, we can flexibly design photon polarization types and wavelength bandwidths. Furthermore, integrating slab and ridge waveguide structures enables device optimization for different quantum interactions.
- High-Efficiency Correlated Photon Pair Generation
We have successfully demonstrated correlated photon pair generation using GaN semiconductor lasers. Generally, GaN lasers have low output power, making quantum light generation challenging. However, we pioneered the world's first adhered slab waveguide using Mg:SLT, achieving high efficiency through strong optical confinement(Figure 2).
Compared to conventional bulk Mg:SLT devices, our design achieves approximately 10 times higher efficiency. Additionally, the device maintains lateral optical freedom, allowing for non-collinear correlated photon pair generation.
This technology provides:- Flexible selection of interaction length
- Expanded wavelength bandwidth of generated photons
- Spatial separation of photon pairs
These improvements enhance the freedom of photon pair characteristics and optical system design.
Our adhered slab waveguide integrates a 3.2 µm periodically poled structure, enabling the realization of a compact, high-efficiency correlated photon pair source operating in the 800 nm wavelength range.
By utilizing Mg:SLT’s superior properties, our system ensures minimal damage and low loss even under 400 nm short-wavelength excitation by GaN lasers. Furthermore, Mg:SLT exhibits high damage resistance across various wavelengths, making it applicable to ultrashort-pulse sum-frequency-generation sampling while maintaining high efficiency and temporal resolution.
Summary
The Quasi-Phase-Matched Nonlinear Optical Devices developed at NIMS have become core components in quantum light control, widely adopted by research institutions worldwide. Various device architectures have opened new pathways for entangled photon pair sources, propelling quantum light generation and control to a new stage.
By enabling battery-powered operation of GaN semiconductor lasers, it is now possible to develop laser-pointer-sized entangled-photon-pair sources. These compact light sources have potential applications in quantum imaging and quantum microscopy, making them valuable tools for next-generation quantum technologies.
TAKAZAWA, Ken
Mechanical device applications of organic single crystal fibers
Overview
It has been reported that some organic crystals suddenly break or hop when they are heated or cooled from room temperature. These sudden crystal motions are attributed to temperature-induced structural phase transitions. The development of molecular actuators by deriving mechanical work from the crystal motion is a fascinating concept. However, the disintegration of crystals upon the phase transitions hampers their device applications.
We resolved this issue by using single crystal nanofibers. Owing to the high flexibility of the nanofibers, they sharply bend instead of break during the phase transition. We succeeded in fabricating fiber-based high-speed actuators using the bending motion. Moreover, we revealed a mechanism of the phase transitions by analyzing the bending motion.
Characteristics
- Nanofibers bend instead of break during structural phase transition.
- The mechanism of phase transition was revealed by analyzing the bending motion.
- Nanofiber-based actuators with high speed and power were developed.
- Force generated by the actuators was large enough to flick an object ~104 times heavier than the fiber itself into the air against gravity.
- Various potential applications.
Major reserch
Single crystals of 1, 2, 4, 5-tetrabromobenzene (TBB) exhibit a sudden jump with disintegration/explosion upon heating to ~40℃. The mechanism behind this effect has been revealed to be a temperature-induced structural phase transition between two polymorphs. The transition causes an accumulation of stress within the crystal, which is then released by explosive motion (left).
We fabricated single crystal fibers of TBB on a substrate by a newly developed method. A TBB fiber repeatedly bent (buckled) and straightened upon heating and cooling, respectively, across the phase transition temperature (center).
The fiber generated the force large enough to flick an object ~104 times heavier than the fiber itself into the air against gravity (right).
Summary
Crystal-based actuators driven by structural phase transition were developed using single crystal fibers of TBB. The fibers could be repeatedly buckled and straightened upon heating and cooling, respectively, at a room temperature range of ~40℃. The force generated by the actuators was large enough to flick an object ~104 times heavier than the fiber itself into the air against gravity. This finding has potential applications in various crystal-based mechanical devices.
OCHIAI, Tetsuyuki
Nonlinear and non-Hermitian nanophotonics
Overview
Photonic crystals, metamaterials, and metallic nanostructures enable us to control the optical properties of materials, light propagation, and light-matter interaction. Optical nonlinearities of constituent materials and non-Hermiticity due to gain/loss and openness further control them at higher levels, and various novel functions such as photo-electric conversion, harmonic generation, low-threshold lasing, anti-lasing, etc. can be realized.
I study theoretically these functions using semi-analytic and/or numerical calculations.
Characteristics
- Ultrafast photo-electric conversion (optical rectification, wireless power transfer, photodetection)
- Novel laser and anti-laser
- Robust photonic integrated circuits with topological effects
Major reserch
Photovoltage in metallic nanoparticles due to optical nonlinearity:
Recently, much interest has been paid to the bulk photovoltaic effect in noncentrosymmetric insulators and semi-conductors, toward solarcell and ultrafast photodetection. In contrast, the photovoltaic effect in metal is dominating on surface, so that nanostructure is very important to enhance the effect. However, physics in metallic nanostructure surfaces has many uncovered aspects due to combined effects of plasmonic resonance, nonlocal response, and quantum spill-out.
Here, we investigated the photovoltage in metallic nanospheres using a semi-analytic approach.
Summary
Amplified spectra in non-Hermite optical systems:
Systems with gain or loss are non-Hermite systems, and recently the non-Hermite skin effect was discovered in which the majority of eigenmodes are localized at the edge in finite systems. We have shown that the effect can be used to design directional lasing and coherent perfect absorption.
Here, as a characteristic analysis of lasing by linear calculations, the amplification factor with respect to the incident light is shown.