- Home
- > Outreach
- > Publications
- > CONVERGENCE
- > ASKING THE RESEARCHER
ASKING THE RESEARCHER
Kazunori Takada MANA Principal Investigator, Nano-Power Field and Unit Director, Soft Ionics Unit
Nano-Power field, Soft Ionics Unit Director, MANA PI
Do all-solid-state lithium ion batteries dream of oxide electrolytes?
Even though we use the same term “lithium ion battery,” the component materials of these batteries are evolving on an almost daily basis. Toward higher power, higher storage capacity and greater safety. The horizon for electrolytes has also expanded from the original organic solvents to the all-solid-state type, and novel materials are being studied. Here, we interviewed Dr. Kazunori Takada, who has spent many years in research on practical application
in view of the requirements of future batteries.
in view of the requirements of future batteries.
The advent of the lithium ion battery
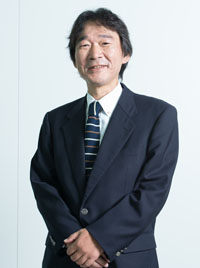
With the popularization of laptop computers and mobile phones, secondary cells, and particularly lithium ion batteries, have become a necessity of life in the modern age. Their range of applications is also continuing to expand, as seen in onboard batteries for hybrid vehicles, large-scale storage batteries in smart grids for efficient use of solar power, etc.
However, when Dr. Takada finished graduate school, joined a private-sector company, and began research and development on solid electrolytes, there was still no prospect that solid-state cells would become a reality. “I joined the Central Research Laboratories of Panasonic Corporation (then Matsushita Electric Industrial Co., Ltd.) in 1986 and began research on solid electrolytes. Among solid electrolytes, the object of research at the time was electrolytes that transport copper ions and silver ions, which have comparatively high ion conductivity. However, since their ionization energy is small and we cannot take out an adequate voltage, I was doing research on the possibility of using those materials in ion devices rather than in secondary cells.”
Later, Sony introduced commercial lithium ion batteries in 1991, and the world’s attention was focused on battery development. Lithium ion batteries operate by movement of lithium ions back and forth through an electrolyte which electrically joins the positive electrode and negative electrode. Other secondary types of cells, for instance, nickel-metal hydride batteries, those cells can only produce a voltage of 1.2 V because an aqueous solution is used in the electrolyte. Among lithium ion batteries, which aim at high energy density, voltages of 3.5-3.7 V have been obtained in commercial products by using an organic solvent in the electrolyte, and voltages of approximately 5 V have been obtained at the research level.
However, when Dr. Takada finished graduate school, joined a private-sector company, and began research and development on solid electrolytes, there was still no prospect that solid-state cells would become a reality. “I joined the Central Research Laboratories of Panasonic Corporation (then Matsushita Electric Industrial Co., Ltd.) in 1986 and began research on solid electrolytes. Among solid electrolytes, the object of research at the time was electrolytes that transport copper ions and silver ions, which have comparatively high ion conductivity. However, since their ionization energy is small and we cannot take out an adequate voltage, I was doing research on the possibility of using those materials in ion devices rather than in secondary cells.”
Later, Sony introduced commercial lithium ion batteries in 1991, and the world’s attention was focused on battery development. Lithium ion batteries operate by movement of lithium ions back and forth through an electrolyte which electrically joins the positive electrode and negative electrode. Other secondary types of cells, for instance, nickel-metal hydride batteries, those cells can only produce a voltage of 1.2 V because an aqueous solution is used in the electrolyte. Among lithium ion batteries, which aim at high energy density, voltages of 3.5-3.7 V have been obtained in commercial products by using an organic solvent in the electrolyte, and voltages of approximately 5 V have been obtained at the research level.
A breakthrough toward the realization of all-solid-state batteries
However, because the organic solvents used in lithium ion batteries are flammable substances, the realization of all-solid-state batteries, which use a solid in the electrolyte, is desired from the viewpoint of safety. Moreover, since solid substances with high ionic conductivities have been discovered in recent years, solid electrolytes are now regarded as the more reliable materials. However, in the battery as a whole, high output power cannot be obtained unless accompanied by the high ionic conduction between the materials, that is, between the electrolyte and electrodes, the electrolyte interface and grain boundaries, and so on.
“A substance with strong oxidizing power is required in the positive electrode of a battery, and a substance with strong reducing power is needed in the negative electrode. Since substances with totally different natures are joined, barriers to ionic conduction appear at the interfaces between the electrolyte and the electrodes. In order to eliminate these obstacles, I developed a nanosized buffer layer which is inserted in the interface.” In this way, Dr. Takada succeeded in realizing high output by inserting a new buffer layer between a sulfide solid electrolyte and an oxide electrode, and thus achieved a breakthrough toward practical application of all-solidstate lithium ion batteries.
“Originally, I was told to use lithium cobalt oxide (LiCoO2) in the oxide positive electrode in an all-solid-state lithium ion battery by my superior when I was in the company, but I didn’t act on that advice immediately because I didn’t think that a positive electrode which exhibits a potential of as high as 4 V would function with a sulfide solid electrolyte. However, a colleague tried assembling a battery and it worked well. Of course, the output was not high enough, but since it had been said solid-state batteries inherently have low output performance, I didn’t pay it that much attention.”
Although Dr. Takada’s workplace at the time was searching for commercial products, he moved to NIMS while the problem of output performance was still unsolved. “After I moved to Tsukuba and built cells using various materials, I also found materials with high output performance, and conversely, it came to seem that the high resistance of the LiCoO2 positive electrode was anomalous. I realized that, after all, thinking that an anomaly occurs at the interface between a high potential positive electrode and a sulfide solid electrolyte is more natural. In the end, it took me 10 years to come to that realization, but after that, it took a relatively short time to increase output.”
As a result of the development of a buffer layer with a thickness of about 5 nm, which eliminated the barrier to ionic conduction at this interface, the output characteristics of all-solid-state lithium ion batteries became comparable to those of commercially-available lithium ion batteries. Today, the Nano-System Computational Science Group at MANA is continuing research to elucidate the mechanism of this phenomenon.
“A substance with strong oxidizing power is required in the positive electrode of a battery, and a substance with strong reducing power is needed in the negative electrode. Since substances with totally different natures are joined, barriers to ionic conduction appear at the interfaces between the electrolyte and the electrodes. In order to eliminate these obstacles, I developed a nanosized buffer layer which is inserted in the interface.” In this way, Dr. Takada succeeded in realizing high output by inserting a new buffer layer between a sulfide solid electrolyte and an oxide electrode, and thus achieved a breakthrough toward practical application of all-solidstate lithium ion batteries.
“Originally, I was told to use lithium cobalt oxide (LiCoO2) in the oxide positive electrode in an all-solid-state lithium ion battery by my superior when I was in the company, but I didn’t act on that advice immediately because I didn’t think that a positive electrode which exhibits a potential of as high as 4 V would function with a sulfide solid electrolyte. However, a colleague tried assembling a battery and it worked well. Of course, the output was not high enough, but since it had been said solid-state batteries inherently have low output performance, I didn’t pay it that much attention.”
Although Dr. Takada’s workplace at the time was searching for commercial products, he moved to NIMS while the problem of output performance was still unsolved. “After I moved to Tsukuba and built cells using various materials, I also found materials with high output performance, and conversely, it came to seem that the high resistance of the LiCoO2 positive electrode was anomalous. I realized that, after all, thinking that an anomaly occurs at the interface between a high potential positive electrode and a sulfide solid electrolyte is more natural. In the end, it took me 10 years to come to that realization, but after that, it took a relatively short time to increase output.”
As a result of the development of a buffer layer with a thickness of about 5 nm, which eliminated the barrier to ionic conduction at this interface, the output characteristics of all-solid-state lithium ion batteries became comparable to those of commercially-available lithium ion batteries. Today, the Nano-System Computational Science Group at MANA is continuing research to elucidate the mechanism of this phenomenon.
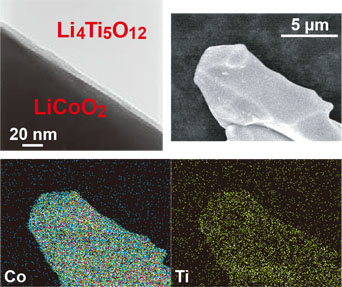
Electron microscope images of the LiCoO² particles that formed the buffer layer. An enlarged view of the buffer layer region (upper left), and an image of a particle (upper right). The images at the bottom show the distributions of Co and Ti investigated by energy dispersive X-ray spectroscopy.
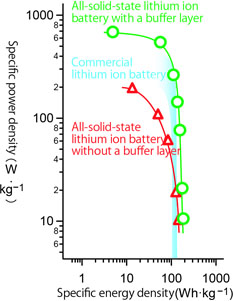
Output characteristics of an all-solid-state lithium ion battery with a buffer layer.
Study of oxide electrolytes and nanoarchitectonics
Although sulfides are thought to be the only favorable substance in this type of solid electrolyte at present, many problems still remain to be overcome.These include process-related restrictions on the use of sulfides due to the fact that sulfides are extremely sensitive to the atmosphere and cannot be handled in air, as well as problems when a battery is damaged and the sulfide leaks out, among others.
Therefore, advancing a step forward, Dr. Takada is now devoting the greatest effort to research aimed at using oxides as solid electrolytes. Based on their crystal structures, three oxides which are suitable for solid electrolytes are known. The NASICON type and perovskite type, which were already known in the 1990s, and the garnet type, which was discovered more recently, were studied. “The ionic conductivity in the crystal structures of all of these oxides is 10-³ S/cm, and there is no problem from the viewpoint of ion transport, but movement is poor due to their extremely high resistance at grain boundaries, or at the interface at points in contact with the electrolyte. We have to do something about that.”
“For me, and for batteries, this interface is nanoarchitectonics. The space charge layer at the interface, which is said to have a thickness on the order of 10nm, this often controls ion transport in a battery. We have to focus our attention on that,” Dr. Takada emphasizes. Because the quantity of electricity that can be stored in electrode particles is proportional to the volume of the particles, use of large particles is advantage for storing energy. Although the volume of a particle is proportional to the third power of its diameter, the surface area through which ions can enter and leave a particle only increases by the second power of the particle diameter. Accordingly, as the size of the particles increases, it becomes increasingly important to create an interface through which ions can enter and leave at high speed. “Battery materials usually have a micrometer size, but what determines the transport phenomenon of ions entering and leaving the crystal structure is this nanosize region. I am now considering various ideas, for example, a new buffer material for oxides, in order to control this space charge layer. I want to overcome this problem and realize an electrolyte using an oxide.”
Therefore, advancing a step forward, Dr. Takada is now devoting the greatest effort to research aimed at using oxides as solid electrolytes. Based on their crystal structures, three oxides which are suitable for solid electrolytes are known. The NASICON type and perovskite type, which were already known in the 1990s, and the garnet type, which was discovered more recently, were studied. “The ionic conductivity in the crystal structures of all of these oxides is 10-³ S/cm, and there is no problem from the viewpoint of ion transport, but movement is poor due to their extremely high resistance at grain boundaries, or at the interface at points in contact with the electrolyte. We have to do something about that.”
“For me, and for batteries, this interface is nanoarchitectonics. The space charge layer at the interface, which is said to have a thickness on the order of 10nm, this often controls ion transport in a battery. We have to focus our attention on that,” Dr. Takada emphasizes. Because the quantity of electricity that can be stored in electrode particles is proportional to the volume of the particles, use of large particles is advantage for storing energy. Although the volume of a particle is proportional to the third power of its diameter, the surface area through which ions can enter and leave a particle only increases by the second power of the particle diameter. Accordingly, as the size of the particles increases, it becomes increasingly important to create an interface through which ions can enter and leave at high speed. “Battery materials usually have a micrometer size, but what determines the transport phenomenon of ions entering and leaving the crystal structure is this nanosize region. I am now considering various ideas, for example, a new buffer material for oxides, in order to control this space charge layer. I want to overcome this problem and realize an electrolyte using an oxide.”
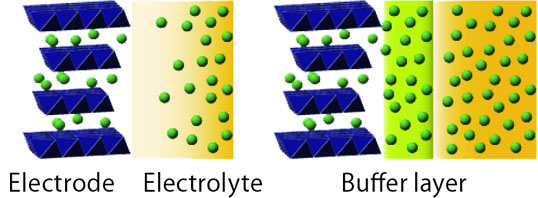
The space charge layer of the interface which controls ion transport in a battery.
Novel techniques for material development began to turn toward batteries
In the development of batteries, various components, including the materials of the electrodes and the electrolyte, their structures, etc. are interrelated in complex ways. In this, Dr. Takada, who has constantly focused on solid electrolytes, says that the highest priority in that research is output. “What’s more, this means increasing output as an essential material property. Although there is an apparent increase even when particles are reduced to the nanosize, I think they can only be adjusted for the first time when considering the application. Requirements are diverse, depending on the device, for example, ranging from the thin-film battery of an IC card to large-scale storage batteries for smart grids. If it is possible to increase performance by interfacial control of the battery materials, it will then become possible to design the particle size and structure, the electrode materials and other specifications suited to the application. For this reason, my primary aim is to develop battery materials with intrinsically high performance.”
Dr. Takada is affiliated with a large number of organizations, including MANA, of course, and also the Battery Materials Unit in the Environment and Energy Materials Division at NIMS, the NIMS-Toyota Materials Center of Excellence for Sustainable Mobility, and the NIMS Open Innovation Center, among others. He has a real feeling that specialists in various areas of fundamental research have turned their attention toward batteries in recent years. “I feel that new techniques for material development for realizing next-generation batteries are appearing. For example, development of analytical techniques, and computational science are more closely related than in the past. Since energy problems are now influencing every field of research, research related to batteries is also increasing dramatically.”
“I’m busy every day, but I play soccer to relieve the stress. I’ve been playing since I was in junior high school,” Dr. Takada laughs.
Dr. Takada is affiliated with a large number of organizations, including MANA, of course, and also the Battery Materials Unit in the Environment and Energy Materials Division at NIMS, the NIMS-Toyota Materials Center of Excellence for Sustainable Mobility, and the NIMS Open Innovation Center, among others. He has a real feeling that specialists in various areas of fundamental research have turned their attention toward batteries in recent years. “I feel that new techniques for material development for realizing next-generation batteries are appearing. For example, development of analytical techniques, and computational science are more closely related than in the past. Since energy problems are now influencing every field of research, research related to batteries is also increasing dramatically.”
“I’m busy every day, but I play soccer to relieve the stress. I’ve been playing since I was in junior high school,” Dr. Takada laughs.
Kazunori Takada Kazunori Takada MANA Principal Investigator, Nano-Power Field and Unit Director, Soft Ionics Unit
Nano-Power field, Soft Ionics Unit Director, MANA PI
Nano-Power field, Soft Ionics Unit Director, MANA PI
Completed the first half of the doctoral course at the Osaka University Graduate School of Science specializing in physics in 1986, and joined the Central Research Laboratories of Matsushita Electric Industrial Co., Ltd. (now Panasonic Corporation) the same year. Received his Ph.D. (Engineering) from Osaka City University in 1991. Joined the Technology Laboratory of Matsushita Battery Industrial Co., Ltd. in 1991, and entered the National Institute for Research on Inorganic Materials,
one of the predecessors of NIMS as a Special Researcher in 1999. Dr. Takada currently has a number of affiliations, including Unit Director of the Battery Materials Unit in the Environment and Energy Materials Division, NIMS. He has also participated in MANA since its inception in 2007 and has been a MANA Principal Investigator since 2008.