NIMS Award 2025 Winners have been selected!
This year’s selection focused on the field of environmental and energy materials, with the aim of honoring exceptional achievements pertaining to “energy-related materials and technologies that pave the way toward a sustainable society”. In addition to pioneering the research field of perovskite solar cells, the awardees were responsible for incorporating a critical element, the solid-state hole transport layer, which led to a dramatic improvement of the cell’s stability and photoelectric conversion efficiency. The series of work has enabled this technology to come a long way toward practical application and was recognized as a truly global achievement.
The ceremony and recipients’ lectures for the NIMS Award 2025 will take place during the NIMS Award Symposium, to be held on Tuesday, November 11th at the Tsukuba International Congress Center.
Development and commercialization of “perovskite solar cells"
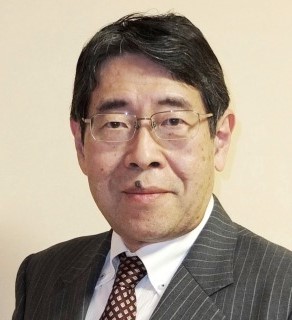
Prof. Tsutomu Miyasaka
Professor of Engineering,
Toin University of Yokohama
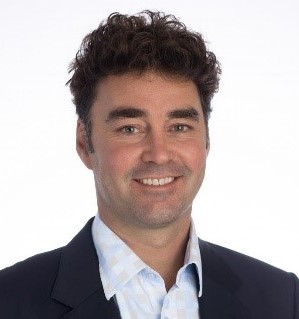
Prof. Henry J. Snaith
Professor of Physics,
University of Oxford
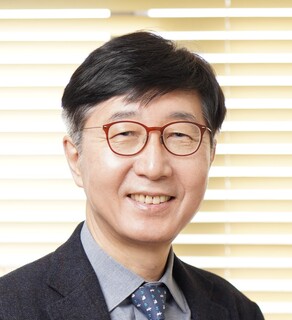
Prof. Nam-Gyu Park
Professor of Chemical Engineering, Sungkyunkwan University
Research Summary and Impact on the Academic and Industrial Sectors
Research Summary
Prof. Miyasaka was the first in the world to apply lead halide perovskite semiconductors—known for their high absorption coefficient in the visible light spectrum—to solar cells, opening a new research field for perovskite solar cells. The device design initially followed a wet-type similar to that of dye-sensitized solar cells, using an iodine-based electrolyte solution as the hole transport layer. This posed a stability issue, however, as the perovskite thin film would dissolve in the electrolyte. Later, through a collaborative effort between Prof. Snaith and Prof. Miyasaka, and in parallel with Prof. Park’s independent research, a solid-state perovskite solar cell was developed using Spiro-MeOTAD as the hole transport layer. This innovation dramatically improved both stability and photoelectric conversion efficiency. The series of breakthroughs served as a major catalyst for global interest in perovskite solar cell research. Since then, numerous universities and companies worldwide have joined the race to develop this technology.
Impact on the Academic and Industrial Sectors
Research and development efforts are underway across many countries to scale up the surface area of perovskite solar cells and enhance their long-term reliability. Unlike crystalline silicon, the material used in the conventional silicon solar cells, which requires a high-temperature processing at around 1400°C, perovskite solar cells can be manufactured at a much lower temperature of approximately 100°C. This low-temperature process allows for production on lightweight and flexible substrates such as plastic. Researchers are also actively pursuing the development of tandem solar cells to utilize the solar spectrum more efficiently; since the band gap of perovskite materials can be adjusted by changing their composition, they positioned a perovskite on the top cell and materials, including crystalline silicon, CIGS, and GaAs, on the bottom cell.
In Japan, private companies ranging from major chemical manufacturers to start-ups are leading the charge in perovskite solar cell development. In addition to showcasing their technologies at the 2025 Osaka Expo, they have taken advantage of the lightweight and flexible nature of the perovskite devices to begin pilot installations in places where the use of silicon solar cells had been impractical. These companies have also initiated trial sales.
NIMS Award Symposium 2025
NIMS Award Ceremony and Commemorative Lecture
at Tsukuba International Congress Center
November 11th, 2025