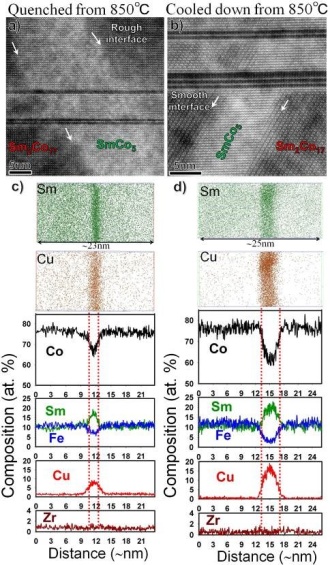
Fig. 1. (a) and (b) STEM-HAADF image and (c)
and (d) 3DAP atom maps of Sm and Cu and
calculated composition profile obtained from
the Sm2Co17-type magnets quenched and slowly
cooled down from aging temperature of 850℃ [3].
and (d) 3DAP atom maps of Sm and Cu and
calculated composition profile obtained from
the Sm2Co17-type magnets quenched and slowly
cooled down from aging temperature of 850℃ [3].
Commercial Sm(Co0.784Fe0.100Cu0.088Zr0.028)7.19 sintered magnets with two different heat treatment conditions, one quenched rapidly and the other slowly cooled from 850℃ were studied. The sample slowly cooled down from 850℃ showed the high coercivity of 2.6 T, while coercivity of the quenched sample was only 0.14 T. Figure 1 (a) and (b) show high resolution STEM-HAADF images obtained from the quenched and slowly cooled samples respectively. Sm2Co17 matrix phase, SmCo5 cell boundary phase, and Z-phase are observed in the microstructure. Unlike the quenched sample, SmCo5/Sm2Co17 interface is sharp and smooth in the slowly cooled sample. Figure 1 (c) and (d) show 3D atom maps of Sm and Cu and their composition profiles obtained from the two different cell boundaries of the quenched and slowly cooled samples. Enrichment of 8.6 at. % of Cu and 7.7 at. % Fe was found in the cell boundary of the quenched sample while the SmCo5 cell boundary phase of slowly cooled down sample contains 15.4 at. % of Cu and 3.0 at. % Fe. In addition, the distribution of Cu broader than that of Sm was found in the cell boundary of the quenched sample. Micromagnetic simulations showed that the enrichment of Fe in the cell boundary and the broad distribution of Cu results in a smaller gradient of K1 through the 2:17/1:5 interface, which decreases the pinning strength of the cell boundary phase. This explains the low coercivity in the quenched sample.